Building Science and the Laws of Thermodynamics, Part 2
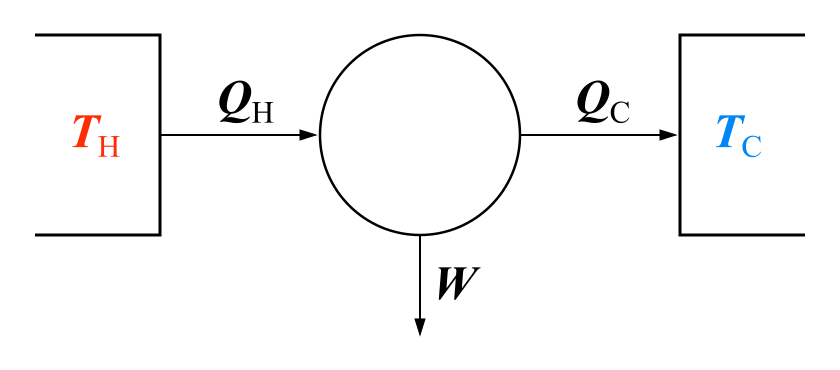
The first law of thermodynamics is one of the more well-known laws of physics, especially in its generalized form of conservation of energy. It provides us with the power to see through stupid ideas and scams. It’s so important, in fact, that even Homer Simpson invokes the laws of thermodynamics when Lisa violated the first law:
But the first law led to a crisis of sorts as scientists looked into it further.
The second law of thermodynamics
The problem was that the first law of thermodynamics would allow all manner of bizarre things to happen. As we did in the previous article, let’s consider a glass of lukewarm water. It would not violate the first law for the water in the bottom half of the glass to get cold, possibly even freezing into a block of ice. For that to happen without violating the first law, the water in the top part of the glass would have to get hot, maintaining the same amount of thermal energy in the glass.
Have you ever seen that happen? No. It may not violate the first law, but we know from our own direct experience that things like that just don’t happen. That’s where the second law of thermodynamics comes in. This one is a bit more complex and has several, equivalent, formulations. We could geek out over heat engines, Carnot efficiency, entropy, and the arrow of time, but for the purposes of building science, the statement of the second law by Rudolf Clausius is the one of most significance:
Heat can never pass from a colder to a warmer body without some other change, connected therewith, occurring at the same time.
In winter, the heat from a fireplace naturally wants to move to the outdoors. Hot to cold. Heat in the outdoor air doesn’t spontaneously make its way into our house “without some other change.” We can get heat from the outdoor air to heat our home with a heat pump, but that piece of equipment has other stuff going on and doesn’t violate the second law. But even then, when you look at how it really works, heat still flows from the warmer to the cooler object. (For more details, see my article on how a heat pump gets heat from cold.)
Heat moving from hot to cold is the form of the second law of thermodynamics I alluded to in the first paragraph of the first article of this series. This meaning of the second law, as well as its application to the flow of moisture (wet to dry) and air (high pressure to low pressure), is taught in many introductory building science classes.
Safety in numbers
A powerful way to understand the second law of thermodynamics comes from statistical mechanics. That glass of lukewarm water, for example, contains gazillions of water molecules. We’re talking lots and lots of zeros. Multiples of Avogadro’s number, which is:
602,000,000,000,000,000,000,000
Each one of those molecules has a particular velocity and position at any given time. The potential combinations of molecular states yield almost incomprehensibly large numbers. The reason we don’t see that lukewarm water separate into a hot section and a cold section is that the odds are against it. Way, way, way against it.
Shirts, pants, and friends
Here’s an example that might help you understand the power of large numbers. Let’s say you have five shirts in five different colors. That’s five “states” you can be in. You can go to work or school each weekday wearing a different color shirt. Or, assuming you do laundry each evening and dress in the dark the next morning, pulling a shirt randomly from the drawer gives you a one in five chance of wearing the red shirt on any given day.
Now, if you also have five pairs of pants in those same colors, the number of days you can go without repeating your outfit is much greater. For each pair of pants, you’ve got five different shirts so you have a total of 5 x 5 combinations. You can go 25 days without repeating an outfit. Or, if you dress in the dark, now there’s one chance in 25 you’ll wear your red shirt and red pants on the same day.
Expanding the example, if you have a friend with the same set of clothing options, she also has 25 possible combinations. For each of your 25 combinations, she could pick any one of hers. The total number of combinations here is 25 x 25, or 625. There’s one chance in 625 that you’ll both show up dressed all in red if you both choose your clothes randomly. That’s not a likely outcome, but it could happen. Right?
Let’s grow the group to five people now, each with five different shirts and five different pairs of pants. For each person we add to the group, we multiply by 25 again. The number of possible combinations is now nearly 10 million! If you all choose your outfits randomly, there’s only one chance in about 10 million that you’ll all show up dressed in red shirts and red pants. (But wouldn’t you stand out if you did!)
If we expand again to everyone in the world, all 7.6 billion of us, I think you can see that that all-red possibility is never gonna happen.
The punishing power of large numbers
Going back to that glass of lukewarm water again, the probability of getting separation of temperatures is far less likely than that of 7.6 billion people randomly picking red shirts and pants on the same day. We’re talking numbers of particles that are on the order of 10 to the 23rd power now, not just 10 to the 9th power. With numbers this large, the overwhelming majority of states would yield uniform temperature throughout the glass.
The second law states that heat never moves from a colder to a warmer object. The statistical mechanics version of it says that it could happen. It just doesn’t because the odds in its favor are infinitesimally tiny.
Building science applications
The second law is referenced widely in building science. To have any hope of designing building enclosures that work, we need to know which direction heat, air, and moisture are likely to flow. In analyzing failures, we can rely on that direction of flow to help us understand what happened. Stack effect, hygrothermal analysis, and energy modeling would be entirely different if we lived in a universe that had the first law but not the second. How would we calculate heat loss and heat gain if we didn’t know which way it might flow?
And then on the mechanical equipment side, the heat engine formulations of the second law carry great weight. They say we can’t get 100% efficiency from a heat engine (which turns heat into work) or a refrigerator (which uses work to move heat). The equation for the Carnot efficiency even gives us a way to check the claims of efficiency for such devices. All we need to know are the two temperatures it operates between, the sink and the source temperatures.
Ginsberg’s Theorem
Here’s kind of tongue-in-cheek version of the first, second, and third laws of thermodynamics:
- You can’t win.
- You can’t break even.
- You can’t get out of the game.
I’ve heard this many times but just today found it’s attributed to the poet Allen Ginsberg and called Ginsberg’s Theorem.
The plight of the universe
Going just a wee bit beyond building science, the second law of thermodynamics implies that the ultimate fate of the universe is heat death. Given sufficient time, all the energy of the universe will end up spread out uniformly, with everything at the same temperature. That assumes the universe is an isolated system, of course. If our universe is simply a speck of matter in some larger universe, our fate may depend on what happens there, much as an ant’s fate might depend on which way that kid points the magnifying glass.
As you can see, the second law is arguably the most interesting of the laws of thermodynamics.
Allison Bailes of Atlanta, Georgia, is a speaker, writer, building science consultant, and founder of Energy Vanguard. He is also the author of the Energy Vanguard Blog and is writing a book. You can follow him on Twitter at @EnergyVanguard.
Other Articles in This Series
Building Science and the Laws of Thermodynamics, Part Zero
Building Science and the Laws of Thermodynamics, Part 1
Building Science and the Laws of Thermodynamics, Part 3
Related Articles
Why Doesn’t Heat Flow Backwards?
How the Heck Does a Heat Pump Get Heat from Cold?!
What IS Heat Anyway? – Building Science Fundamentals
NOTE: Comments are moderated. Your comment will not appear below until approved.
This Post Has 7 Comments
Comments are closed.
Allison, I agree that the
Allison, I agree that the second law is the most interesting, but you don’t seem to be getting much response. I guess that turkey is more interesting. But I have to object to this post. Why can you quote the Simpsons but I can’t quote The Big Bang Theory? I guess that the man with the gold makes the rules . . .
I have another quiz. Has
I have another quiz. Has anyone ever heard of a Ranque-Hilsch Vortex Tube? If not, it is a mechanical device with no moving parts and no work input that is supplied with compressed air. It then divides the compressed air into a hot outlet air stream and a cold outlet air stream. They are sold commercially and used for spot cooling applications. I actually bought one and used it for demonstrations in the classroom in my teaching days. You can easily get 30 degrees of temperature change. Why is this not a violation of the Second Law? I will let Allison explain how it works since he probably knew Ranque or Hilsch!
The simple answer is that the
The simple answer is that the Ranque-Hilsch Vortex Tubes are enchanted. The complex answer is that each employs a clone of Maxwell’s Demon, who stands at the entrance to the tube and, simply sorts the incoming air molecules according to their velocities, thus producing hot and cold streams, which can be directed to cool or heat objects at opposite ends of the tube.
Here is a great quote about
Here is a great quote about the 2nd Law that I got from a Super Bowl Commercial this past Sunday:
“You don’t have to know about Plato and Aristotle to serve. You don’t have to know Einstein’s theory of relativity to serve. You don’t have to know the second theory of thermodynamics in physics to serve. You only need a heart full of grace, a soul generated by love.”
Who do you think said this? It wasn’t Maxwell. To give you another hint, this quote was obviously made before the Second “Theory” became “Law” 😉
Also, it was quoted to encourage you to buy a certain brand of pickup truck.
Question is why does my
Question is why does my ground source heat pump system work so well to heat and cool my 6,000 sf home? I average $156 per month over a 7 year period. No outside fan noise for a compressor and home is very comfortable with 3 units a 2 ton, 3 ton and a 4 ton sounding like a refrigerator when running. I have 6 bore holes 300’ deep with 1” HDPE plastic pipe fused together. Even our football coach has a system in his new home. Why does it work so well using energy from the ground and not the air?
Gerald, since no one else
Gerald, since no one else seems to want to help, I will take a shot at answering your question using the Second Law.
If you look at the diagram at the top of this blog and reverse the arrows so that it represents a heat pump instead of a power cycle, the Second Law says that the maximum COP for this system in heating is COPHmax = QH/W = TH/(TH-TL). TH is the indoor temperature, TL is the heat “source” temperature, so TH-TL is the difference between the indoor temperature and heat source temperature. Thus, the smaller the value of TH-TL, the greater is the possible value for COPH. I am not sure where you live, but if it is in the northern U.S., TL is probably around 50 F if the ground is your heat source and TL is usually below 50 F if the outside air is your heat source. Thus, a ground-coupled heat pump should win whenever the outdoor temperature is below 50 F. The same logic applies to cooling where COPLmax = QL/W = TL/(TH-TL), and TL is now the indoor temperature and TH is the outdoor air or ground temperature. As long as the ground temperature is below the outdoor air temperature, a ground-coupled heat pump wins. I hope that your saved energy pays for your six 300-foot bore holes.
I am not criticizing your choice, in fact, I use to own a house with a ground-water source heat pump and I loved it for all of the reasons you mentioned. It was a relatively cheap system since it used the same 80-foot deep well and pump as the water supply for my house, and then just dumped the water in a shallow field tile in my back yard with sandy soil.
Greeting from New Zealand. I
Greeting from New Zealand. I just want to thank you for this blog. I love your down to earth explanation and it definitely seems like you are having fun along the way. I wish more teaching resources were like this. I really love the energyvanguard blog, keep up the good work.