Open-Loop vs. Closed-Loop Ground Source Heat Pumps
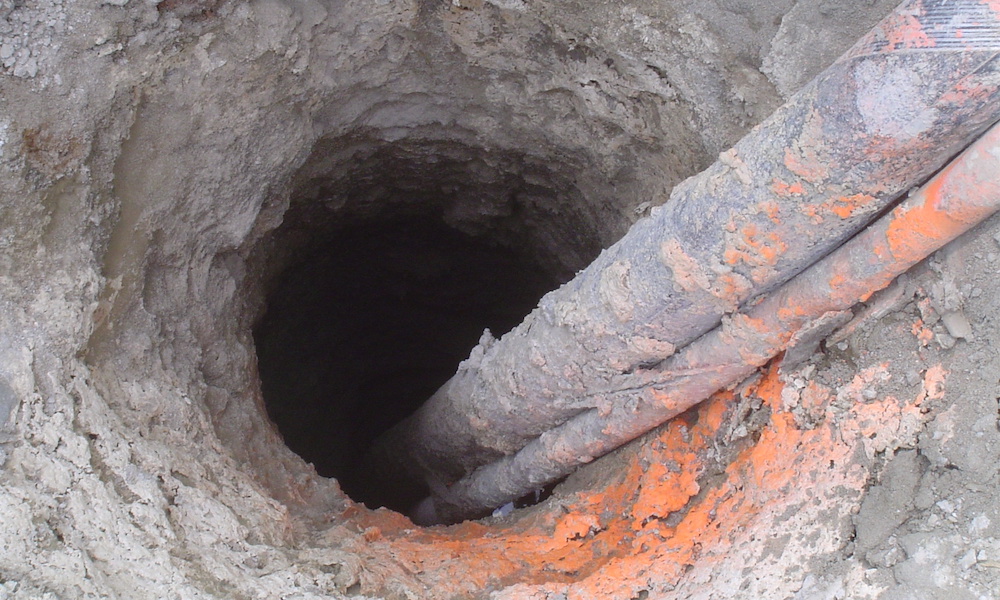
I mainly talk about air source heat pumps here. But ground-source heat pumps are an important type of heat pump, too. So today, let’s look at the issue of open-loop vs. closed-loop design when installing this type of heat pump.
What is a ground source heat pump?
First, I use the term ground source heat pump, but they’re also called ground-coupled or geothermal heat pumps. They do exactly the same thing as air source heat pumps: They move heat between indoors and outdoors. The equipment is really just a heat exchanger with a lot of interesting physics that keeps your house cool in summer and warm in winter.
The only difference is that in a ground source heat pump, the outdoor exchange happens with the ground, groundwater, or surface water. The diagram below shows four different ways that outdoor heat exchange can occur.
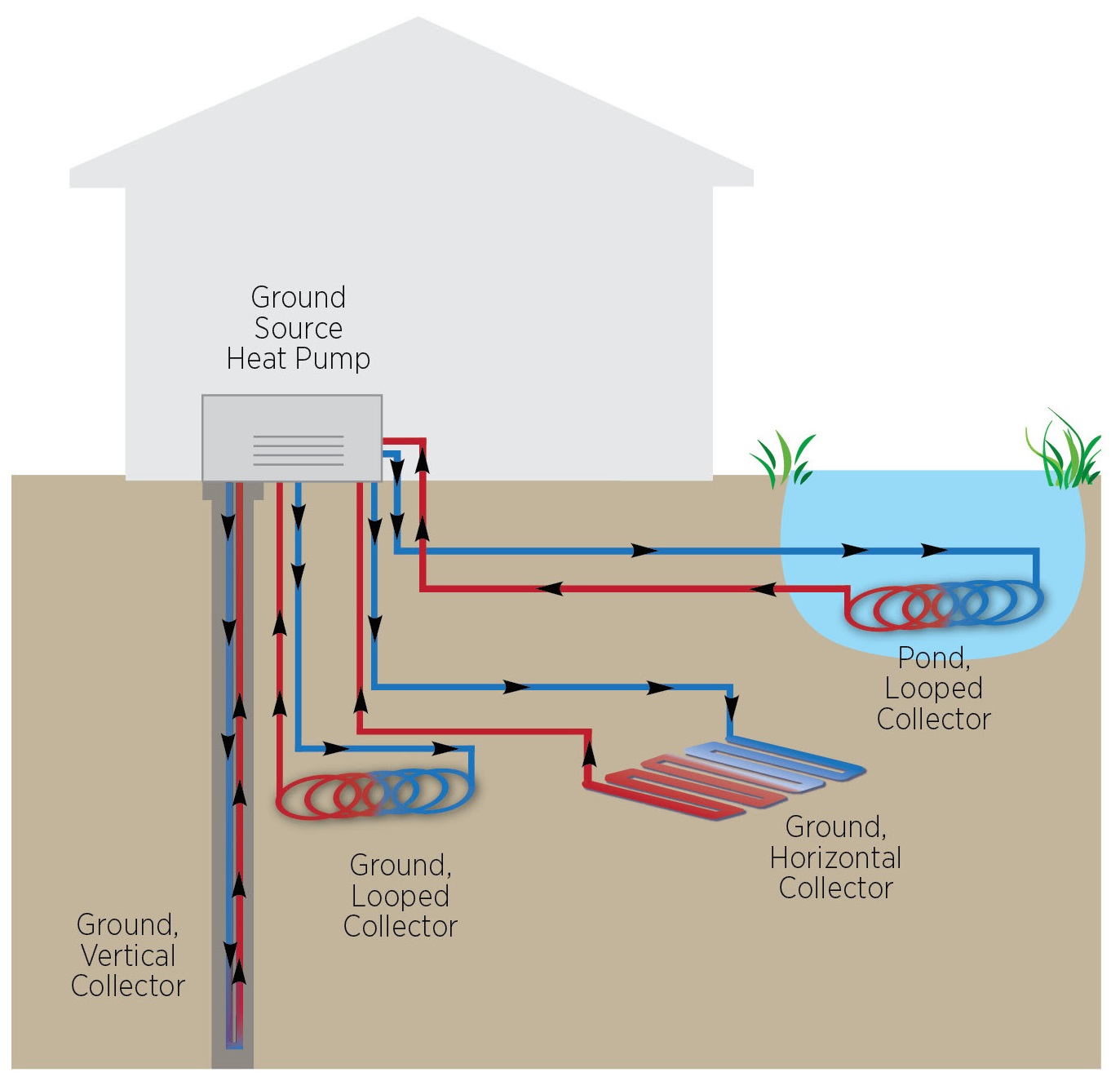
Open-loop vs. closed-loop
Anytime you’re in the position of choosing a heating or cooling system, you have to make decisions. One that comes up with ground source heat pumps is deciding between the open-loop vs. closed-loop types for the outdoor heat source or sink. The diagram above shows four closed-loop systems.
In that type, the fluid that exchanges heat with the ground is a mixture of antifreeze and water. As the name suggests, it’s contained in a closed system that circulates the fluid through the pipes. That same fluid going through the pipes over and over.
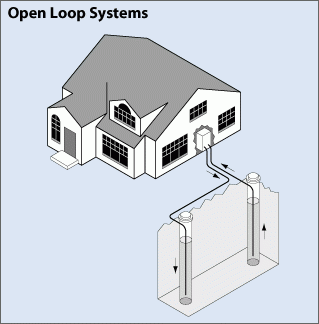
But there are three other types of open-loop systems that handle the water responsibly after the heat exchange. The diagram above shows one of them, the doublet. It has water being pumped out of one well and then sent back into a different well. The other two open-loop types are the standing column well and the dynamic closed loop. The latter is really an open-loop/closed-loop hybrid. See Jay Egg’s article on this topic for more detail.
Pretty simple concept, right?
Which type is better?
As with most things, which type is better depends on whom you ask. But there is a pretty good agreement among a lot of people in the industry that one type is better than the other. Before I tell you which it is, though, let’s list the important issues.
- Water quality
- Efficiency
- Groundwater contamination
- Aquifer depletion
- Legality
Let’s start at the end because the last three are connected. In some places, you’re not allowed to install an open-loop system (or certain types of open-loop systems anyway). In dry areas out West, water is scarce and highly regulated. Aquifer depletion and groundwater contamination can make that problem worse.
An open-loop system can pump A LOT of water from the ground. In a large, inefficient house, that could be many tens of thousands of gallons per year. Groundwater contamination happens when the used water just gets dumped onto the ground. There it can carry surface contaminants down into the ground.
That leaves the issues of efficiency and water quality from the list above.
Efficiency
On the surface, open-loop systems seem to be more efficient than closed-loop systems. One reason for that is that water transfers heat more readily than the closed-loop antifreeze mixture because it’s more conductive. Also, if you have a large supply of groundwater, you’ll have a more constant temperature over the course of the heating or cooling season. The area around a closed-loop system can heat up in summer and cool down in winter, making it harder to cool or heat the house.
But there’s more to it than that. I spoke with John-Paul Kiesel of Water Furnace, one of the biggest names in ground source heat pumps. He told me that when you look up the efficiency of the two types of systems, you’re not getting the full picture. It’s like comparing apples to oranges. That’s because a significant source of energy use is absent from the open-loop efficiency.
The closed-loop system efficiency includes the pump energy whereas the open-loop efficiency does not. And pump energy can be much higher in an open-loop system.
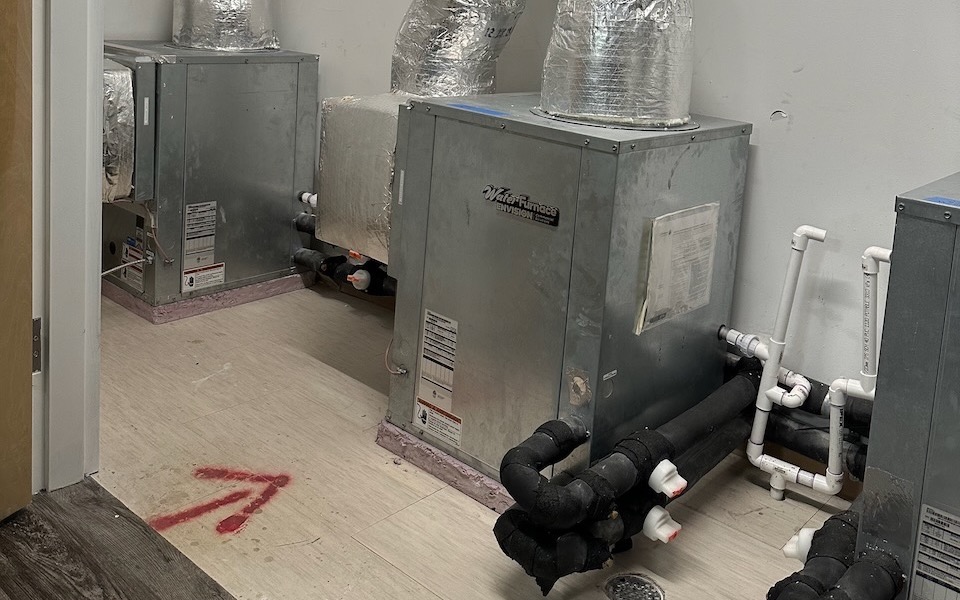
Let’s say you have matching systems that are of the vertical well type. The open-loop system works against gravity the whole time as it pulls water from the ground. The closed-loop system, however, is pulling the antifreeze mixture up one pipe while the fluid is going down the other side. The fluid going down helps push the other side up, resulting in less pump energy use.
Kiesel said about the efficiency question, “If you have a properly designed closed loop, it shouldn’t make any difference.”
Water quality
The quality of the water in an open-loop system is what can make a huge difference. You’re putting groundwater or pond water in contact with the heat exchanger. That can lead to pitting, corrosion, scale, or other problems. The acidity and mineral content of the water as well as the amount of silt and even iron bacteria can shorten the life of the equipment.
Kiesel told me that the expected lifetime of a closed-loop system is 25 to 30 years. With poor water quality, an open-loop ground source heat pump may last only 10 to 15 years. He also told me he doesn’t have a preference for open-loop or closed-loop. As with just about everything in the world of building science, both types can work well when designed, installed, and commissioned properly.
Comments from industry pros
I posted a question on LinkedIn about this topic to see what others had to say. I especially wanted to hear from those with direct experience with either or both types. Here are some of the comments I got there:
Bradford White (engineer): “I’m definitely in the closed-loop camp as a default. Control your water, know your water.”
Ty Branaman (HVAC instructor): “Closed loops require purging but less contamination and less energy usage in the pump since what is pushed is pulled in the loop.”
Rob Brown (former contractor, now working for a manufacturer): “Open loops are FAR more susceptible to failure.”
John-Paul Kiesel (manufacturer’s rep): “Open loop works great as long as the water quality is in range and flow rates are adequate.”
Dan Nall (architect): “I’ve had bad experiences with the 2 open loop systems with which I’ve been involved. An open loop system is sort of like having unprotected s3x with a relative stranger.”
Ross Trethewey (engineer): “We have designed 250+ geothermal heat pumps systems in the northeast over the last 15 years and every single one is a closed loop system.”
The sum and substance
There’s your quick overview of open-loop vs. closed-loop ground source heat pumps. One thing I didn’t mention is cost or ease of installation. For both of those, the word on the street is that the open-loop system wins. It’s just easier and cheaper to install a pump-and-dump system. But the drawbacks, especially the water quality issue, can eat into any savings you get upfront if the equipment lasts only half as long. As always, do your homework, get a full design upfront, find a good contractor to install it, and then get it commissioned.
Afterword
My description of open-loop ground source heat pumps above just scratches the surface. To understand the intricacies of it better, see this article by Jay Egg:
When Does Aquifer Thermal Energy Transfer Work Best?
Allison A. Bailes III, PhD is a speaker, writer, building science consultant, and the founder of Energy Vanguard in Decatur, Georgia. He has a doctorate in physics and is the author of a bestselling book on building science. He also writes the Energy Vanguard Blog. For more updates, you can subscribe to our newsletter and follow him on LinkedIn.
Related Articles
A Bit of Confusing Heat Pump Terminology
3 Types of Heat Pumps and Air Conditioners
What Is a Mini-Split Heat Pump?
Comments are welcome and moderated. Your comment will not appear below until approved.
This Post Has 53 Comments
Comments are closed.
Thank you for calling it a GSHP and not stressing Geothermal like the industry does so often. Even the EPA puts GSHP on the same page as true geothermal, after just changing their name from geothermal heat pumps. It’s so confusing. https://www.epa.gov/rhc/geothermal-heating-and-cooling-technologies
I agree with Lloyd. Geothermal is a misnomer for this type of system and should only be used for systems that are getting really hot water from deep in the earth. I am not even crazy about GSHP, since the ground is only a “source” in heating. During cooling, the ground is a “sink”, so I guess the “S” could work either way. I prefer “ground-coupled heat pump” (GCHP) since that is a better general description for a reversible system.
And I agree with you, Roy, for agreeing with Lloyd who agrees with me! :~)
I also agree with you about ground source vs. ground coupled, but for some reason I keep saying ground source. Maybe it’s time to change that.
Lloyd: I think we’ve lost that battle, but I’m not going to give up the fight.
The first energy-efficient home I built in 2003 used an open-loop ground source heat pump. The slime from iron bacteria in the well clogged the small diameter tubes in the HP heat exchanger in about three months. After replacing the fouled HP heat exchanger, I used my mechanical engineering skills to design and build an intermediate heat transfer system: A closed-loop antifreeze system feeding the HP heat exchanger and running through a larger, easier to clean/replace heat exchanger between the groundwater and the antifreeze. It worked well, but I am sure there was some loss in efficiency.
I never specified an open loop ground source heat pump in any subsequent projects.
Rick: I learned about iron bacteria from the house I built the same time you built yours. I didn’t have ground coupled heat pump, but I did have a well that got contaminated. Our dirty water filters were always an orange-red as a result.
My issue with these is primarily a cost one when compared to a good air-source solution. Burying that line seems to cost a good bit more than you would think. Of course, it has been a few years since I looked at this option.
Norman: I don’t have good data on cost comparison between air coupled and ground coupled heat pumps, but what I’ve been told is that the federal tax credit in the US makes the cost difference mostly go away.
You say that the life of a closed system is 25 to 30 years.
What wears out? The pump? The heat exchanger? The compressor? The loop?
The major expense of a closed loop system would be drilling of the well or digging of the trench with the loop install. As long as the loop doesn’t fail and need replacement, replacement of the rest of the system should be a relatively low cost.
Do we need to worry about the loop failing?
Installed my closed loop ground source heat pump in 1993. The loop had a 50 year warranty.
Lived in the house for 27 years, had no issue with loop. Original Hydro Heat Unit was replaced with Waterfurnace when original unit was 18 years old. Original unit had on going refrigerant leaks, mainly at reversing valve welds.
David: I’m not a contractor or manufacturer, but maybe some who have experience with what fails can answer that question. Regarding the “relatively low cost” of equipment replacement, though, I think a lot of people might not find it so low. Replacing any heating or cooling equipment runs thousands of dollars.
Allison: My current home is in Texas and I had to replace the air coupled heat pump a few years back. It was about 20 years old and not cheap.
OK, I’ll rephrase the question: How does the cost of the GSHP compare to the cost of an air coupled heat pump – excluding the cost of the loop?
I don’t have access to consumer pricing for air-to-air or air-to-water heat pumps, but I do have some knowledge of the manufacturing costs. An air-to-water heat pump should be somewhat cheaper to manufacture than a complete air-to-air split system (indoor and outdoor unit). That may not be the case today due to the economies-of-scale of the two types of systems, but if the GCHP market grows, they should become more cost competitive in terms of the equipment itself. So the major difference in installed cost will be mainly due to the ground loop itself, not the equipment.
I agree that a closed system is generally better than an open system for the reasons given. However, I had an open system in upstate NY in the 1990’s that worked great. It used the same well and pump that we used for our potable water needs. The well was only 100 feet deep so pumping power wasn’t a big issue. The heat pump water was just dumped into a short field tile which worked great since our soil was pretty much all sand. The water was also quite clean so fouling didn’t seem to be an issue. I will admit that we only lived there for 5 years, so perhaps there were problems later.
I have to disagree with Allison’s comment that the ground has a higher heat capacity than air. The specific heat of dirt and air are both around 0.2 Btu/lb-F. If you look at specific heat on a per volume basis, dirt is much higher than air due to air’s low density. But neither measure really addresses this issue. When a properly situated heat pump outdoor unit exchanges heat with the air, the air can easily dissipate that heat to the atmosphere via air movement, so the effective heat capacity of the air is essentially infinite. But when heat is transferred to or from the ground, it takes much longer to dissipate since it depends on conduction through a stationary medium. This is why closed ground loops must be properly sized to dissipate that heat throughout the season and from one season to the next.
Roy: Good point about the “effective” heat capacity of the air. Regarding when I wrote “the ground or water that your drawing from or dumping into can have a higher heat capacity than air,” I was thinking more of water, but not every borehole fills with water. And yes, it’s absolutely critical with any type of ground-source heat pump that you understand the geology. The heat exchange can vary widely with type of soil or rock and the presence or absence of water.
I’ll echo the other architect: I also had 2 bad experiences with open loop systems. But they are much cheaper to install than closed so people keep rolling the dice. I did a project with 89 closed loop systems in 2008 and they are still going strong. But as I recall each well was something on the order of 250 ft deep to get the heat exchange for a modest single family home.
There is another type of open-loop GCHP that wasn’t mentioned: A standing-column well. It’s been a long time since I learned about them, but they use a single well, and it is the potable water well. Pump water up from the bottom and return it to the top. The well has to be used for consumption too in order to prevent a significant drop (when heating) or rise (when cooling) in temperature. I believe that is the type of system that Roy C described. This type of system is open-loop so the pump has to provide enough pressure to overcome the piping and heat exchanger pressure drops AS WELL AS the “lift” from the upper surface of the water column to the end of the return pipe, if the level drops below the return pipe end. If the water level in the well-column drops significantly (as from a drought or overdrawing of the aquifer), the pump would need more “head” to overcome that.
I think the best way to think about the more common closed loop well in which there is a down and up pipe with a U-bend at the bottom, is like any closed loop hydronic system, like the heating systems that are common in the northeast. There is no pumping head from lifting the water in a closed loop. The mass of water on either side of the pump is the same. All the pump has to do is overcome the pressure loss of of the piping and the heat pump heat exchanger for the flow rate desired.
Gene: I just updated the article with a mention of the standing column well and also the dynamic closed loop. That info came from an article by Jay Egg, a ground-source heat pump expert in Arizona, which I’ve also provided the link to above.
Allison,
Have you heard about “Waterless” ground source heat pump systems?
https://waterlessgeothermal.com/
I would be careful about these “direct” GCHP’s. They require copper pipe instead of plastic which is much more expensive. They require much higher refrigerant charges and refrigerant is becoming increasingly more expensive. On top of that, soon you will be required to use flammable refrigerants where there are charge limits based on safety that may prevent the use of these direct systems.
I will admit that copper is a much better heat conductor than plastic, but the limiting thermal resistance in most closed loop systems is the ground itself.
Roy – you are exactly correct about the future of refrigerants. Two states, MN and ME, have recently approved overly-broad regulations that ban the sale of all PFAS materials by 2032 and 2030, respectively. These PFAS materials include all fluorinated refrigerants including the most recent low-GWP products. If these regulations are not stopped or amended, we’ll be back to ammonia, alcohol, or other dangerous, less-efficient alternatives within a decade. At least 10 other states are considering the same PFAS regulations.
Gene: My system just dumped the used heat pump water into a shallow tile (perforated tubing) in the ground near my house, much like the field tile for a septic tank. It did not return the water to the well. I believe that many, if not most, places do not allow dumping water of any kind back into the aquifer. There is just too much risk of contamination if the system fails. In my case, the rejected water gets filtered by 100 feet of sand before it gets back to the aquifer, same as my septic system.
RE cost of ground vs air heatpumps. On one of the ground source manufacturer’s web sites there’s an energy use calculator where you plug in the house’s specifics and it tells you the energy usage difference of ground vs air, calculating in any SEER you wish to input. As the structure gets better, the difference gets smaller. The cost of the wells being the major difference, on an Energy Star house of 2000 sf, I calculated 25 years for the payback for ground vs air. If you own a large, old, drafty house that can’t economically be insulated, then ground is worth it. If it’s smaller, new and tight, air is the winner.
Allison – Here in North Central PA, the use of GSHP expanded 25 years ago because of the inefficiencies of air-to-air heat pumps in cold climates when outdoor temperatures went below 25-30F. With the advent of new air-to-air systems that work efficiently in single digit outdoor temperatures, do you see the demand for GSHP decreasing?
Chiming in – I do expect demand for GSHP to decrease owing to cold temperature ASHPs. My summer travels took me to North Carolina high country, far down east Maine, and southern Ontario. Inverter / variable capacity cold weather inverter heat pumps are proliferating in all those places.
If you are using Air Source heat pump efficiency figures to calculate what heating would cost based on how much fuel your current heating system used and it’s efficiency, you should note that the US-DOE has come up with a new Heating System Performance Factor called HSPF-2. It is 15% lower than the old HSPF was. So for example, a multi-zone split ASHP that typically had a SEER of 10.3 kBtu / kWh HSPF is now rated 8.75 kBtu / kWh, delivering 15% less heat than we used to think they did They did this because the old value consistently UNDER-estimated the HP operating cost. the SEER was also modified to SEER-2 in kBtu/kWh, but for cooling it is only 5% worse than the previous SEER values. Both of these adjustments make ground coupled heat pumps look better, but their installation cost is still a big barrier. I am interested in Air-to-Water heat pumps. They are less likely to leak high GWP refrigerants with no field-made mechanical tube joints, and provide better heat distribution by reusing existing hydronic piping common in the northeast. If we could get US makers to switch to propane refrigerant so that they could make 170F hot water for baseboard heat, they would have a great electric-powered boiler replacement.
There are air-to-water heat pumps available today with nonflammable refrigerants that can be used to replace boilers today. Manufacturers would be making propane units if there was a market for them, but there won’t be unless the government bans our safer refrigerants for various environmental reasons.
Note that a hydronic system using an air-to-water heat pump will be significantly less efficient than a forced-air system using a heat pump because of the higher supply temperature required for hydronic systems. I think a lot of hydronic heating systems will be replaced with forced-air systems if and when fossil fuels are banned.
A problem with propane as the refrigerant in comfort cooling system arises from fire safety. The risk of just several ounces of extremely flammable propane leaking out of a small to midsize chest freezer (I’ve seen new chest / display freezers wearing R-290 stickers at food stores) seems fairly minor. I believe the max charge by weight allowed for propane is just 5 ounces owing to the flammability problem. Self contained cabinet refrigerators have all their joints sealed in factory conditions and with (I presume) rigorous testing after assembly, and components are all housed / protected by cabinetry.
A typical split comfort cooling system is charged with 2-3 pounds of refrigerant per nominal ton, and typically has at least 6 joints brazed in the field by installers whose skill, integrity and procedures vary widely. These joints, copper tubes, etc are exposed both indoors and out.
Stated another way a 3-4 ton split heat pump system would have a refrigerant charge weighing anywhere from 6-12 pounds. I’m horrified of even the slightest possibility of that amount of propane (~half a BBQ tank) engulfing my home’s interior owing to a joint or component fail on the indoor side – lines, air handler coil, metering device, manifolds, etc.
Then there’s the risk of poorly trained installers improperly servicing systems containing large amounts of propane – service procedures often include brazing out failed components and brazing in replacements…what could POSSIBLY go wrong?
From my days on the water I remember a weather rule of thumb: “Red sky at night, sailor’s delight, red sky in morning, sailors take warning” When I worked the water as a cop, I learned an addition “…red sky at noon, something went boom!”
I am not an engineer, but my understanding is that propane is a more effective refrigerant, so the charges are about half as big, with a legal limit of 2.2 pounds. Also, most of the units are designed as “monoblocs” totally sealed at the factory, and you just pipe water to the interior. No special training is involved in installing them.
I wrote an article about this, website link above.
Curt: You are right, there are a lot of safety concerns with using propane as a refrigerant in our current “direct” systems. If we get forced to this “natural” refrigerant, it will have to be an indirect system with a packaged (factory-sealed) air-to-water heat pump located outdoors and a secondary loop using water or antifreeze to transfer heat to or from an indoor unit. The safety standards for this type of system are under development.
Yes, Curt, propane is a flammable refrigerant, but natural gas is flammable too and quite a few of us have it running to several points of use inside our homes, often in an iron pipe network connected with threaded joints. Some folks are a little jittery about that, but most are not. In a sense the “safe” non-flammable synthetic refrigerants we are using are really “time-bombs” which are heavily promoted as the answer to decarbonization, but have GWPs 2,085 times worse than the CO2 produced from burning natural gas. One leak from our mini-split (5 lbs of R-410A) is equal to a year’s worth of burning natural gas for heat and hot water, and the newer R-32 is better, but still terrible with a GWP = 750. Mini-splits with flare-nut tubing connections (4 per indoor head) are prone to LEAK. We have had 2 in 5 years. Those refrigerants cost well over $100 / pound to replace and IMHO are setting us up for our next climate disaster.
I don’t know any North American Air-To-Water heat pump makers for the residential market who are using propane, and only one, Chiltrix, is using R-32, but the seven European producers of ATW heat pumps I know of are using propane. Their units can make 170F hot water (vs 130F max for our R-410A or R-32 models) and so are able to directly replace hot water boilers. The ATW heat pumps are factory sealed hermetic units with brazed refrigerant lines less likely to leak and no propane indoors. I am more concerned about all the R-410A leaking into the atmosphere than I would be with a small charge of propane outside my house. ATW heat pumps making 170F hot water can directly replace boilers with baseboard or radiators for heating, but would still need fan-coils to get enough sensible cooling capacity, and the latent cooling would have to be done separately, since the existing heating system is not design to remove moisture (but would condense moisture in all the wrong places if supplied with chilled water). But latent cooling could be done with a stand-alone dehumidifier or with new fan-coils with new insulated piping. The latter seems feasible in the unfinished basements of 2 or 3 story homes. If propane was used as a refrigerants in mini-splits with flare-nut connections indoors I would be fearful of a leak too, but in Air-To-Water heat pumps the propane remains confined outdoor in a hermetic factory-sealed unit, so I think the flammability and leak risks are acceptable.
There are many other advantages too, like back-up resistance heat, ease of monitoring performance with a temperature-pressure gauge, installation can be done by plumbers with years of boiler experience, virtually zero GWP if it does leak, ability to heat domestic hot water, better heat distribution (all rooms), outdoor unit can be quickly removed for repair offsite, and when the heat pump does reach the end its life, the outdoor unit can be replaced while the indoor distribution system is reused. With mini-splits the whole system has to be replaced. Since they can make 170F hot water, unlike mini-splits they can recover from night setback, to maintain the heating savings you get with your setback thermostats.
ATW heat pumps with propane refrigerant seem to me to have many advantages over mini-splits with current high GWP refrigerants. I don’t understand our lack of concern for the leak problem if we expect to convert our entire housing stock to this kind of system. I think the ducted central heat pumps with all-brazed joints are less prone to the leak problem, although less efficient than mini-splits and difficult to apply in older homes without ducts.
Gene, I agree with most of your points, but I am not clear about your comments about using fan-coils for cooling. If you are using a reversible ATW heat pump for heating and cooling, you can get latent capacity in the fan-coil during cooling just like we do with refrigerant fan-coils in ducted systems today. If you are going to put in a ducted fan-coil for cooling, you might as well also use it for heating since you can then get by with lower temperature supply water which will improve the heating efficiency. In this case, I would not try to use the ATW system for domestic water heating–I would just go with a separate heat pump domestic water heater.
Here in hot humid north Florida we do install ground source heat pumps, but only in very limited circumstances. Historically, I’ve “unsold” many more GSHP systems than I’ve sold. By that I mean I steer most typical inland sales leads I receive back to conventional air source systems.
Why? The complexity and first cost overwhelms the marginal efficiency improvements over air source in Florida. Air source systems have steadily become more efficient especially with the onset of variable capacity heat pumps. Our deep ground temperatures (mid 70s or higher) reduce the efficiency of closed loop systems since entering water temperature of those tends to be 10-20*F higher than deep ground temperature…which in turn compares poorly to average summer outdoor air temperature. The temperature at which heat is rejected drives most of the efficiency of any and all vapor compression AC systems.
On the subject of online calculated savings over air source I’ll not name names, but one I worked with for awhile often promised outlandishly high savings…sometimes on a par with the prospective customer’s ENTIRE electricity bill…a complete non-starter that contaminated many leads.
Where DO we deploy GSHP? At the beach. There I can reasonably forecast better payback of GSHP over ASHP simply because the salt laden air at oceanfront homes corrodes ASHPs to death far more quickly (2-3x) than normal 15-ish year lifetime of those systems inland. GSHP compressor cabinets can (and we insist they be) installed indoors typically in a garage or mechanical room out of the weather.
Owing to elevated ground water temperatures, we deploy open loop with reinjection – two bores to a deep aquifer ideally 50+ feet apart. That saves the closed loop temperature penalty. We minimize pump power by specifying a variable speed pump. As one can imagine, all that can easily run $25-30k just for ground water, so the upfront cost is easily 2x ASHP systems.
I don’t deny that GSHPs may be a better fit up north where they benefit from 45-60*F deep ground temperatures and are sometimes able to eliminate annual consumption of 500+ gallons of wildly expensive trucked propane or heating oil, but with some air source heat pumps now able to deliver rated capacity at as low as -13*F, the operating cost advantage compared to ASHP is narrowing fast.
Final note – Allison described open loop pump and dump systems as using “many tens of thousands of gallons per year” Consumption can actually easily run to a MILLION gallons per year: A 3 ton system consuming 2 GPM per ton that operates 3000 hours per year (in my area, 2000 cooling, 1000 heating) pencils out to 1,080,000 gallons annually. Some may quibble with some of my numbers, but it’s in the ballpark…that’s a LOT of water!
I live in the Caribbean and have been exploring installing a closed loop system in my beach house, cooling cost are stupid expensive here so I plan to couple this with solar, I’m about 300 meters from the beach and there’s a river close by, I have no idea what ground temps below 150 feet will be and there’s no research. What is your opinion on ground temps around me, when air hover around 28-34 year round?
A rough rule-of-thumb for deep ground temperatures is that it is near the average annual air temperature. One of the problems with GCHP’s in the hotter climates is that you are constantly putting heat into the ground and heating it up over time, especially if you undersize the ground loop, thus performance degrades. In a heating-dominated cold climate, the opposite occurs–the ground around the loop keeps getting colder. The ideal application is in the intermediate climates where the annual heating load is roughly equal to the annual cooling load. In this case, you are putting heat in the ground in the summer and taking it out in the winter so that you have no cumulative heating or cooling of the ground during subsequent years.
Of course that’s 28-34 Celsius.
Roughly speaking, deep groundwater temp somewhat tracks average annual air temp, so based on that, maybe 30*C in your area. If true you’d size and specify equipment based on entering water temperature around 40*C.
My opinion matters little – you need data. Look for an opportunity to measure water temp from a nearby well. If you intend a shallow closed loop system, such as trenches with slinky tubes, that may be vulnerable to slowly building up heat over multiple seasons especially since your climate requires no heating.
This is a tricky project filled with variables…I’m not sure I’d want to be involved in “Serial No. 1” if there are no similar systems in your area.
I thought about boring 4 holes separated about 40 feet each,150 feet deep with 1” Pex to account for heat concentration, wells near caves in rocky areas here have chilly under 55F measures in studies done by French scientist a while back, but like I said I’m located in a beach area with no caves around that’s why I’m wondering about underground temps but I’ll try to measure a neighbor’s well if he allows me, thanks for your input.
Don’t guess at loop field dimensions, tube lengths, diameter, etc. Closed loop water source heat pump installers use software and data from site-specific conditions to size and configure loop fields.
RoyC, I’m thinking about the problem we have in older northern cities where we have millions of existing homes with hydronic heat. If we can get a propane Air-to-Water heat pump that makes 170F hot water we have an easy path to electrifying heating, and plumbers can install it. But cooling with that old distribution system is more difficult. So how can we do it without tearing up the interior walls to use the existing piping system to distribute chilled water?
We could add low wall fan coils in the existing baseboard piping, but that would only work for sensible cooling, with a chilled water supply temperature of about 60F, that is, above the air dew point we want for comfort. We have to remove the moisture another way to do a simple conversion without a major renovation to the home’s interior to install insulated piping suitable for 45F chilled water. Of course once we install the fan coils we don’t really need the 170F hot water any more, but a lower water supply temperature will only make the heat pump more efficient. But to avoid condensation under every baseboard or even in the walls where pipes are concealed, we cannot dehumidify with these new fan coils; the existing piping won’t allow that.
So we would have to do that another way. One simple way is just to use a dehumidifier and control to the rh we want. One unit should do the job since moisture from everywhere in the house will migrate to its cold coil because of vapor pressure differences. Another way to do the latent cooling, if you have access to the basement ceiling, is to run new insulated home-run lines to new fan coils on the first floor (ducted or not), which you can do without damage to the first floor walls, and supply them with 45F chilled water from the heat pump. The return water from those units would be about 55F and could be mixed with warm return water to 60F and supplied to the old piping system for sensible cooling without danger of condensation.
I think that asking homeowners in these old hydronically heated homes to install mini-splits with their potential for climate damage due to refrigerant leaks, high repair costs and lack of heat distribution to every room will not only be a hard sell, but will be disappointing to buyers. They do an adequate job of cooling & dehumidifying these old homes, are quiet and efficient, but heat distribution will be worse than in the 19th century when we had fireplaces in every major room. And they cost more to operate than even an atmospheric gas boiler, so there are no savings to entice people to make the switch. Oil & propane customers would see some savings, but not enough to pay for the conversion. Existing hydronic systems do a good job of distributing the heat to every room, so why not use them to do the heating with a heat pump, and with a few additions of fan-coils the cooling too. I admit its a niche problem; most of the US has air-based ducted heating systems, but in older northern cities boilers are very common and we need a solution for them that does not involve major interior renovations or moving out of the house while it is renovated.
There’s a company in the northeast which had intentions of building a system for hydronics, do a Google search for Dandelion.
Gene – Unfortunately there appear to be problems with your approach to electrifying northern homes presently heated with baseboard radiation (inaccurate name – the heat emitters are actually natural convectors)
1) Pumping chilled water into baseboard emitters designed to take advantage of natural convection for heating using water ~100*F warmer than ambient simply won’t work – the natural convection that makes them work well for heating won’t magically reverse itself for cooling.
2) Substituting dehumidifiers in lieu of conventional cooling systems will in nearly all relevant cases decrease comfort owing to the heat emitted by the dehumidification process – approximately 1000 Btu for every pint of water condensed from ambient air plus Watt hours converted to Btuh (1:3.413) consumed by the dehumidification appliance.
3) Any attempt at cooling using a surface chilled below dewpoint will require collecting and draining condensate. You mentioned 60*F as the desired water temperature for sensible cooling via baseboard emitters. Problems with that approach include both very little actual heat transfer from room air to surfaces cooled to 60*F and the risk of uncontrolled moisture whenever air dewpoint exceeds 60*F… a condition quite frequent during hot weather as far north as New England.
Despite the cost, I’m convinced that multizone ductless minisplit heat pumps are the only viable alternative that allows removal of obsolete or failed hydronic systems (baseboard radiation or steam radiators) in older northern and midwestern homes that are not feasibly able to be retrofitted with properly functioning duct systems.
Curt, I agree with most of what you said, but I think Air-To-Water heat pumps can work in existing hydronically heated houses.
1) I agree that the cooling effect with 60F water in baseboards will be small, and that’s why I said we have to add fan-coils for cooling (and for heating if we can’t get the high temperature heat pumps in the US that they have in Europe). But even with fan-coils we can’t get the chilled water down below the dew point because the existing piping and baseboard are not insulated to avoid condensation or handle it.
2) You are correct that the dehumidifier would add sensible heat to the space, but would have the advantage of providing direct humidity control. I found this a link saying “The sensible-to-latent heat removal ratio for a dehumidifier is 50-50 while an air conditioner is closer to 70-30.” [https://unitedcoolair.com/flexible-alternative-portable-environmental-control/ ]. Our dehumidifier consumes 407 watts and if half of that appears as sensible heat added to the space that would be 204 watts x 3.413 Btu/watt-hour =~700 Btu/hr (less than a tenth of a ton), so not too terrible.
3) I agree we don’t want a high space dew point. I was thinking of 75F/50% space condition for a dew point temperature of 55.1F. So we have to keep the chilled water supply to the old distribution system with its combination of old baseboard and new fan coils above that 55F dew point. I propose 60F. We just need to control that dew point, either with a dedicated dehumidifier or with new fan-coils and piping that gets the 45F chilled water supply on the first floor (which could be piped in many basements without too much disruption).
My experience with mini-splits has revealed many shortcomings, and I would not want to rely on one for cold climate heating without a backup (we have two). I think we need a way to electrify our cold weather home stock without a completely new distribution system. We need to be clever about using the distribution piping we already have. It will last the life of the home, whereas the outdoor air-to-water heat pump will need to be replaced about every 15 years.
Unfortunately, I think you may misunderstand the flows and paths of the energy converted and exchanged by a free standing dehumidifier:
1) The entire 407 Watts of electric energy are added to the room as sensible heat (407 * 3.413) = 1389 Btuh. That comes from heat of compression, motor windings, fan energy etc. Every single electrical energy Btu is rejected into the room since the dehumidifier and its air flow is entirely within the room.
2) But wait, there’s more: For the sake of example let me guess that yours is a nominal 30 pint per day model performing at specification. That’s 1.25 pints removed per hour (condensed from air and drained or stored between tank emptying chores).
In round numbers, the heat transfer needed to evaporate a pint of water is about 1000 Btu. To condense a pint of water from vapor to liquid requires extracting 1000 Btu from the air / water mixture. At 1.25 pints per hour, that’s 1250 Btuh extracted from the room’s air to condense the vapor to water. That heat is extracted by the low pressure (evaporator or “cold side” coil) of the dehumidifier. Where does it go? It is added to the heat energy rejected by the dehumidifier via the high pressure (condenser or “hot side” coil) which explains why the air leaving the dehumidifier is considerably warmer than room temperature.
So the net effect of operating your dehumidifier is the removal of 1250 Btuh of latent heat from the room’s air and the addition of 2639 Btuh sensible heat (1389 from the electricity plus 1250 Btuh converted from latent to sensible via condensing the water vapor).
For simplicity, I’ve omitted the additional heat transfer required to first cool the room’s air down to dewpoint before condensing occurs, but that washes out when the very temporarily cooled air enters the dehumidifier’s high side coil.
I wonder if the 50-50 rule you cite comes from the approximate equality of energy used relative to moisture removed. (1389 Btuh consumed vs 1250 Btuh transferred).
A dehumidifier’s ability to make a cold wet space both warmer and drier is useful when operated in clammy chilly basements in New England, the upper Midwest, and Oh Canada. Pretty much anywhere else the waste heat is a nuisance to be endured or additional load to be rejected by air conditioning systems.
The only way for a dehumidifier to remove heat from a room is to direct its high side condensing coil heat transfer somewhere other than to the air in the room. Window / portable air conditioners do that, as do heat pump water heaters.
This all explains why heat pump water heaters work so well in warm climates – they leverage their modest electricity use (similar to a small dehumidifier) so that all the electrical energy PLUS the sensible heat from the surrounding room air PLUS the latent heat from water condensed from surrounding room air are ALL transferred to the water tank to produce domestic hot water.
I hope this explanation is correct, understandable and helpful…but if not, oh well, the price was right!
Curt, You are correct. ALL the dehumidifier energy does get rejected to the space. I was thinking that the half used to condense the water would end up there, but on reflection, I think you are right. I first thought of the dehumidifier as a solution to separating the latent cooling from the sensible because of a few articles by Joe Lstiburek in their Building America project, in which a low cooling load house had trouble with high humidity and they solved it with a dehumidifier in the hall closed and a louver door, because a whole-house dehumidifier was rather expensive. Maybe a small window AC unit running on low speed would be a better solution, eh? But even so, the 1389 Btu/hr isn’t a huge load.
We have millions of existing homes in the northeast with boilers and baseboard or radiators, and I have been trying to think of a way to utilize their existing hydronic system but convert to a heat pump. The two problems I was struggling with is with the low water temperature of the current ATW heat pumps, the high-temperature heating terminals only have about 30% of their capacity, so would have to be augmented. The second issue is that the old system is not designed to handle chilled water and deal with the condensate. But cooling is an important incentive to conversion, because there will be no cost reduction for gas customers and not enough savings to pay for the installation over it’s lifetime for oil customers. So the only solution I could think of was to add low-wall fan-coils to the existing piping to regain heating capacity and use them for sensible-only cooling, but add something else for the latent cooling. My first thought was a free-standing dehumidifier on the first floor, but then I thought with most of these houses having accessible basement ceilings we could add one or two fan-coils on the first floor piped for 45F chilled water to do the dehumidfying for the whole house.
I have done this on commercial buildings with radiant floors or ceilings, but maybe it’s too complicated for residential. It would probably need a special controller that will always keep the cooling water supply to the old hydronic system above the current air dew point in the house. All those new fan coils will get expensive, but unlike a multi-split air Air-to-Air system, they would not have to be replaced every 15 years when the heat pump fails. They would last the life of the house. Thanks for correcting my thinking on where the dehumidifier energy goes.
John Siegenthaler is an early proponent of ATW heat pumps, but his designs involve new insulated piping home runs to each room’s fan-coil. That works for new construction and single story homes, but in 2 and 3 story homes the damage and disruption caused by the new piping is expensive and may require the occupants to move out for a while. I was looking for a cheaper and less disruptive solution.
I don’t understand why preserving radiators/hot water heat is desirable, unless you’re in a heat-only climate zone. I took all that out of my 1938 house, freed up a lot of floor space and got rid of a source of possible carbon monoxide poisoning. When you walk in my front door, you’d never notice the duct chase unless it’s pointed out.
Galen, I am thinking about how we can decarbonize on a massive scale. I wanted to reuse as much of the current distribution system as possible to keep the cost down. In the northeast’s relative energy cost structure, heat pumps will not pay for themselves in their lifetime. Indeed, gas customers will have higher heating costs. So retaining the existing distribution system reduces the project cost both now and in 15 years when the mini-split fails and both indoor and outdoor components have to be replaced. The existing hydronic system is good for the life of the house and it gets the heat to every room, which mini-splits are not so good at. As Gary A. points out, we need a drop in replacement for a boiler. The Europeans have that with their propane refrigerant Air-To-Water heat pumps that can make 170F hot water, are less likely to leak their refrigerant because they have factory-sealed refrigerant enclosed in the outdoor unit, and if they should leak their GWP is so low that they won’t create a new climate problem, like R-410A will. And the propane is cheap to replace if it does leak, and the unit can be quickly disconnected and repaired in a shop under good working conditions. If we had these high temperature heat pumps here, they could directly replace the boiler by delivering their to a buffer tank indoors.
That’s sort of good-news/bad news to me. The high-temperature heat pump is a drop-in heating solution, but not easily adapted to cooling. I was trying to manage that with the current US ATW heat pumps that operate in the 105-130F range by adding low-wall fan-coils to the existing piping and using them for heating and sensible cooling cooling only, The fan-coils are required to replace the lost heating capacity due to the lower water temperature. The dehumidification would have to be done by other means. So the low temperature ATW heat pumps available today in the US require a lot of interior additions, but still avoid the expense and disruption of tearing out the existing distribution system and opening the walls to put in a whole new insulated piping system. That’s a really nice solution, but with little-to-no cost savings there is no economic motivation to do it, and I don’t think it would ever happen on a mass scale. Their are cheaper ways to get cooling and dehumidification in these older homes. We only really need it for July and part of August and if you have made some insulation upgrades, the biggest issue is dehumidifying in those months. I have heard that Chiltrix is working on a US high-temperature heat pump, and that would be the perfect boiler replacement for both heat and domestic hot water. That has the potential to make electrification likely at a large scale in the northeast where boilers are so common. As Gary A points out, a simple drop-in solution would be a big deal, making the cost competitive with a boiler replacement.
@Gene I think that’s a good strategy. Retrofitting just the heating system is a nice cost-effective approach, and some people even prefer the radiators. But don’t get too caught up on the cooling since most people figure out some sort of work-around for cooling whether it’s window units or a mini-split. In fact, mini-splits are really nice as a back-up like a friend of mine that was able to limp by on the heat from the mini-split for a couple weeks during a cold snap when the radiator lines sprung a leak and it took to get materials for the repair to the primary heating system. A plug-and-play heating solution would be a big deal. Dryers, ranges, and water heaters are relatively simple appliance swaps once one commits to going all electric and does the service and panel upgrades.
Gary, we have a 2-story, 4 bedroom house (<1900 sf) with a condensing boiler, hydronic baseboard heat and a mini-split with one head on each floor (2). The mini-split is acceptable for cooling and humidity control. We can use a few fans to help even out the temperatures. We are pretty well insulated and have shade trees on E, S & W, so even without cooling, on a 95F day we will stay below 82F indoors. That's bearable, but the humidity that comes with it is oppressive. We did have to use our mini-split this winter for heat for about 10 days. Our boiler pulled in enough dirt through its combustion air intake to foul the combustion chamber. I have added a filter box to prevent that from reoccurring. So the mini-split and gas log got us thru that January week until the boiler could be put back on-line. But the heat distribution wasn't as good as the hydronic baseboards.
I read that Chiltrix is working on a high temperature ATW heat pump to solve this boiler replacement problem. I have also heard of one other heating solution not available yet: A microwave boiler; an electric heater for hydronic homes. But it will not have cooling. Maybe mini-splits are the answer for cooling, as you say. Or those new window ACs that wrap over the window sill and allow you to see out and open the window. They are supposed to be getting inverters soon, I have read, so they will be a much quieter and more appealing solution, with a SEER2 comparable to mini-splits. I hear they will be reversible too, for heating.
The flare nut connections at each end of the mini-split's refrigeration lines are their Achilles heel. Four opportunities for a leak for each indoor head. After this winter when we used our mini-split as back up while our boiler was repaired, we didn't use it again until a late spring warm spell. But then it didn't respond. After injecting a dye and half a charge of R-410A it worked for a while, then failed again. After checking all the flare joints they finally found the leak in the upstairs head. The dye and UV light showed a tiny crack in one of the flare nuts; I have it here on my desk. That nut cost $900 to replace including another 2.5 lbs of R-410A. In 5 years of operation we have lost 15 lbs of R-410A to leaks. That is the global warming equal of burning gas for 3 full years in our boiler. So that's why I don't think mini-splits that use R-410A or the somewhat better but still high GWP R-32 are not good solutions to decarbonize our homes in order to deal with the climate crisis. They are too likely to leak and make global warming even worse. Central ducted heat pumps avoid the issue with brazed joints, but are an expensive and difficult solution for older homes without ducts.
There is another issue with heat pumps which I never see discussed. Their low-temperature heat makes recovery from night setback long and difficult (I experimented with 120F water this winter and the recovery took 6-8 hours). Mini-split controls omit night setback scheduling because of this. But night setback reduces the number of heating degree days you have to make up for, so was introduced to save energy and money. You have to give that up with low temperature heat pumps, so we not only pay more than gas for the electricity we use in our heat pump, but we have to supply more energy to maintain occupied temperatures all night. The heat pumps that I hope are coming soon CAN recover from night setback with 170F hot water to baseboards, and then reset to a more efficient lower temperature to maintain that occupied setpoint during the occupied hours. They hold the promise of both setback savings and semi-continuous water circulation for uniform comfort temperature with little fluctuation.
I have a artesian well, that produces 9000 gallons a day, this is my drinking water. The water overflows into my pond, into a ravine and down into the local creek. My thought was always to do a open loop geothermal system with a small circulation pump powered by solar panels. I have lived here for 10 years, water has never stopped and my pond has never frozen over. Is this doable?
I live in south western nys.
Hello: I have had a open loop 5 ton Climatemaster GSHP since 1996. I have a ~ 2800 sq ft house superinsulated with 12″ thick walls ( 9″ fiberglass and 3″ dead aipspace) 9″ fiberglass in second floor ceiling and knee walls. I have to admit, not being and engineer the water loop was done by the seat of my pants. I designed and installed the loop myself. 2 dug wells , one 19′ deep (supply well) & one 12′ deep (return well, ~ 300 feet laterally from my house and ~ 30′ lower in elevation from the house location. The 2 wells are ~ 80 feet apart and I’ve never had a lack of water. The supply well has a 1500 gallon concrete drywell at the bottom and (3) 4′ diameter well tiles stacked on top of it. In the 28 years or operation the system has performed very well. The main service performed was on the zone valve which needs replacement due to wear. ( A side note Taco only sells the replacement power head $$$ but not the replacement valve body $ ). Not sure how often closed loop valves need replacement but I figure they must. The contactor/ relay for the compressor was replaced also. A recent HVAC service check indicated refrigerant was still within specs and the unit was still good to go.
I monitor water temps and plenum temps using original installation performance records as a benchmark. This unit was part of an intro program that gave rebates similar to existing programs. They also performed an efficiciency test to measure the unit against advertised specs.
One parameter I’ve noticed changing is the ground water temp getting colder with the lack of snowcover during our northeast winters.
Relative to the pumping issue mentioned in your article, I observe a siphon effect on the return line which helps pumping efficiency.
Relative to the emergency heat setting, I keep the breaker for the E-heat off. Really don’t need it, I do have 2 propane fireplaces if necessary. I’ve reduced the E-heat size to about 10 kw since I had a backup generator installed. The original ~ 20kw E-heat is as big as my generator and I knew that wouldn’t work well. I’ve never had to go down that road yet, and don’t see it ever happening.
I guess I’ll run this machine in to the ground before replacing it.