Is Low Velocity Bad for Air Flow in Ducts?
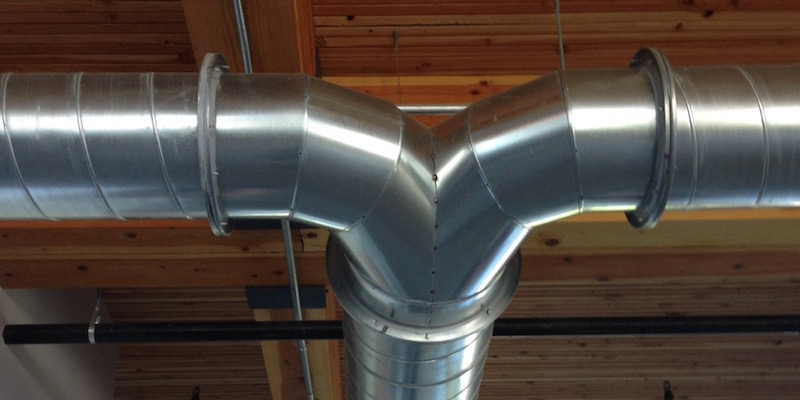
It’s obvious that moving air too quickly through ducts can be a problem. Faster air means more turbulence, more resistance, and more noise. But I run into people who think that low velocity also can be a problem in ducts. Just recently I heard someone talking about how low velocity causes “rolling air” in the ducts. I don’t know what he meant by that (turbulence?), but is low velocity really something we should worry about? And if so, when?
Bigger ducts, lower velocity
I’ve written about the relationship between velocity and duct size before, so let’s do a quick look back at this important principle. The diagram below shows equal volumes of air in two parts of a duct system – one smaller, one larger. What can we say about the air flow in the two places?
One thing we know is that if we have an air flow rate through one part of a duct and no air leaks out or is diverted through another duct, the rate at other parts of the duct must be the same. In other words, if we have 100 cubic feet per minute (cfm) of air flow in the larger piece of duct below, then the air must still flowing at 100 cfm when it gets into the smaller duct. That stems from the Law of Conservation of Mass and the good assumption that air flow in ducts happens at constant density.
If the air flow rate is constant, it’s straightforward to show that the quantity A x v is constant, too. That’s what we call the continuity equation. The simple way of stating this principle is:
As duct size increases, air velocity decreases, and vice versa.
So we can increase the velocity of air in a duct by making the ducts smaller and we can reduce the velocity by making the ducts bigger. But what does duct size do to static pressure drops?
Static pressure drops, equivalent lengths, and velocity
Static pressure drops are the key quantity to worry about with moving air through ducts for a simple reason. The continuity equation tells us that the air flow rate stays constant from one part of a duct to another. But that doesn’t mean the air flow is a constant.
What I mean here is that the air flow rate depends on the resistance to air flow in the duct system, and static pressure is a measure of that resistance. When we reduce the size of a duct or put a fitting in that turns or divides the air or even just move the air through a straight section of duct, we add resistance.
Adding that smaller diameter section to the duct above, for example, increases the resistance and decreases the air flow from what it would have been had we kept the duct the same, larger size. So if 100 cfm of air flows through the configuration above, more than 100 cfm would be flowing if the duct hadn’t gotten smaller.
The more common way of talking about that resistance in ducts is by total effective length (TEL), which is the sum of the equivalent lengths of fittings and the actual lengths of straight sections. And as I’ve discussed before, the equivalent lengths of fittings dominate, as the straight sections add little to the total resistance (unless the straight sections are flex duct not pulled tight).
Now, here’s one more principle we need:
The static pressure drop across any one part of a duct system (fitting or straight section) is proportional to the square of the air flow rate.
And the continuity equation above tells us that the air flow rate is proportional to the velocity. So in a component of constant size (say, a 12 inch diameter elbow), the static pressure drop is proportional to the square of velocity.
I apologize for making you think through a little bit of math here, but hey, we’re not using spherical Bessel functions to solve Schrödinger’s equation in three dimensions. Stick with me a bit longer and this will make you a better person.
Let’s do this with numbers, which always seems to help. Say you have air moving at 900 feet per minute (fpm) through that elbow. The static pressure drop of the air moving through it is proportional to 900 fpm squared. The result of multiplying 900 by itself doesn’t really matter (it’s 810,000) because there’s also a constant of proportionality involved and we’re not going down that path.
We can write this relationship out as:
Δp ~ 900^2
Here, Δp is the change in pressure (or static pressure drop) and 900^2 means squared, or 900 x 900. What really matters here is what happens when we change the velocity. Instead of using 900 fpm, let’s say the air moves through that fitting at half that velocity, 450 fpm. How does that change the static pressure drop? Here’s the answer:
Δp ~ 450^2 = (0.5 x 900)^2 = 0.25 x 900^2
Now we can extract one of the most important lessons of this article:
When you cut the velocity in half, the static pressure drop is cut to a quarter of what it was.
In other words, bigger ducts and lower velocities are better for static pressure than you might have guessed. And guess what? All those equivalent lengths for fittings you can find in ACCA Manual D are based on a velocity of 900 fpm. If you move the air at half that velocity, the actual equivalent length is a quarter of what’s stated in the tables.
How would you like to use a fitting with 10′ of equivalent length instead of 40′? Just move the air through it at 450 fpm instead of 900 fpm and that’s what you get! (Unfortunately, HVAC design software may not make that adjustment for you, using the equivalent length for 900 fpm no matter what the actual velocity is. I know RightSuite Universal doesn’t do it.)
What, Me Worry?
If you’re worried about low velocity in ducts you design or install, take some advice from Alfred E. Neuman.
If it’s throw from the register and mixing in the room that worry you, here are two reasons to relax your mind. First, the velocity of air entering the room is generated by the boot and the register. It’s just like putting your thumb over the end of a garden hose to shoot the water farther in the yard. Second, throw from the register isn’t nearly as important in homes with airtight, well-insulted building enclosures with modern windows that meet today’s building codes. Admittedly, that’s still a small percentage of all the homes out there, but the better the enclosure, the less important throw becomes.
And I’ll give ACCA Manual D the next-to-last word on this topic. Appendix 15 of the Third Edition, Version 2.50 is titled Air Velocity for Ducts and Grilles. Those three and a half pages cover the continuity equation, the benefits of low velocity, balancing dampers, and more. The appendix ends with these words of advice:
There are scores of things to worry about when designing and installing a comfort system. Low velocity through a duct airway is not one of them.
But sometimes you should worry
So I’ve made the argument that low velocity isn’t something to worry about, but that’s not entirely true. Yes, in terms of getting good air flow in the duct, you really can’t go too low. But there’s another important factor besides static pressure that should govern the velocity at which you move air through ducts. And that’s for the next article.
Related Articles
What Happens to Air Flow in Ducts When Size Changes?
Duct Design 3 — Total Effective Length
The Basic Principles of Duct Design, Part 1
NOTE: Comments are moderated. Your comment will not appear below until approved.
This Post Has 47 Comments
Comments are closed.
“Second, throw from the
“Second, throw from the register isn’t nearly as important in homes with airtight, well-insulted building enclosures with modern windows that meet today’s building codes. It’s just not.”
Yes and no…while I agree that we no longer need worry as much about cold / hot / drafty single pane windows…we do need to be concerned that new energy efficient homes may need just 1 ton of HVAC per 1200 – 1800 or even higher square feet.
Since for proper humidity control we limit HVAC system air flow to 350-400 CFM per ton, we have to pay proper attention to fully circulating / mixing the air in the much larger volumes served per ton of HVAC
Curt, I think I partially
Curt, I think I partially agree with you. Mixing on a large scale is important. You can’t just heat half of the house or one floor of a two-story. But most residential A/C units only do two jobs, thermal and humidity control. In a tight, well-insulated building, neither is all that important, and natural forces like convection and radiation will do most of the work for us. As far as air quality, no amount of mixing is going to have the effect on air quality that source control has. And the mixing that is needed for air quality purposes is not going to be efficiently provided by the HVAC system. Most of the time I see new tight homes use small diffusers to achieve the throw they need from low-flow ducts. So overall I tend to agree with the premise. The only exception would be for HVAC systems that provide outdoor air ventilation to occupied areas for air quality purposes.
Now you’ve done it Allison.
Now you’ve done it Allison. The next thing I will be reading is the path with the greatest equivalent length may not be the one with the greatest pressure drop. Surely you know that most people are more comfortable with opinions they are most familiar with. Paradigm shifting is just not popular these days. Perhaps you should think about reinforcing some strangely held myths to create esteem and surety.
I am fond of, “I always put 3 or 4 lbs. of extra freon in the unit just in case there is a leak. You can never have too much.”
Curt, As inverters become the
Curt, As inverters become the new norm, I am just about convinced we can soon toss accidental humidity control from an HP/AC out the window. Even IF they maintain a consistent SHR at lower frequencies, 25% of 3,800 BTUH is not going to satisfy the more consistent latent load in these homes. AND ERV’s don’t help. Remember that latent loads don’t follow outdoor temperatures like sensible.
The sad thing is, we don’t know it because no one is tracking humidity. Those that want to are stuck with archaic instrumentation that can be off as much as 10%-12% RH. Very frustrating.
> Even IF they maintain a
> Even IF they maintain a consistent SHR
Which inverter based mini-splits typically don’t do. Fan output doesn’t drop nearly as far as compressor output. So as you need a greater percentage of latent removal (lower SHR), SHR actually rises to ~1.0.
Also note that 350 CFM/ton only applies to the airflow over the coil. Any amount of airflow bypassing the coil is OK – so the total CFM/ton through the ducts can be anything higher.
Many of us have seen
Many of us have seen empirical evidence that lower cfm in ducts will work without serious consequences, even though it does reduce velocity in existing registers. This is often demonstrated with retrofits using two to five stage residential equipment with existing ductwork sized for one stage. Often the older homes had excessive static pressure at full cfm anyway, so the lower stages may be more energy efficient, run longer and are certainly quieter with the variable speed blowers. Air balancing would seem to be a concern as a two stage unit ramps from full cfm to perhaps 67% flow. Ideally a fully modulating zone damper system such as available from Trane, Carrier and Jackson Systems would help resolve the balancing and velocity issues.
“The sad thing is, we don’t
“The sad thing is, we don’t know it because no one is tracking humidity. Those that want to are stuck with archaic instrumentation that can be off as much as 10%-12% RH. Very frustrating. ”
Once again, yes and no –
We make it part of our business to track and attempt to control humidity. I have a “white paper” of sorts that lays out 20 contributors to indoor humidity and solutions to mitigate / eliminate them.
Most of our conventional HVAC installs include a thermostat and air handler able to actively dehumidify (but, of course, only in the course of sensible cooling)
I do agree that sensor accuracy can be an issue. I carry a pair of Field Piece humidity “sticks” that function acceptably.
Skill with humidity sets us apart from the 1000+ licensed HVAC entities in our market.
Allison, you are touching on
Allison, you are touching on a topic that should have been exposed so many years ago. And you almost get to the flash point regarding this topic, but only bump it slightly. I suspect you know where to pull your punches to keep the peace, unlike me who does not know any better. So here goes, very brief at first and see what happens.
I agree and teach that bigger ducts (lower velocities) significantly reduce airflow resistance and perhaps to the extent that we do not need to worry so much about good fittings and bad. I teach that WHEN you have a high velocity, such as a cramped return air drop carrying the total airflow at 900 fpm, use a good fitting. But IF you can maintain a low velocity, quit worrying about the fittings and a few extra 90s. Or another way to say this, if you have a long run, simply up-size the duct to lower the velocity, and the resistance to that room is minimized.
And now the flash point idea. I have always disliked “friction rate”. I can’t measure it. It’s hard to explain to a class, and now, with the help of blogs like this, I might add it is 100% uncalculatable and therefore of very little if any value. Why? Friction rate is derived by using available static pressure and total effective length (TEL). TEL cannot be calculated because the “effective” length is fully, and significantly, affected by velocity, which changes and varies throughout the system. But friction rate is so ingrained in Manual D and our duct calculator. It seems most everyone in the business is faithful to this useless number but I think a much simpler duct design method needs to evolve from this topic. It’s amazing how well a distribution system works if it is simply large enough to minimize resistance. (Yes, I’m aware we seldom are given this much room, but this is just science talk for now.)
So, here’s my new duct design class: Up-size ducts, minimize resistance, deliver adequate volume, then damper and balance. Class is over.
Well said Dan !
Well said Dan !
For me it is more about how
For me it is more about how quickly I can cool a room, and also AC efficiency. The more air you move across the coils, the higher the efficiency of the unit. I know most people don’t care about how fast a room can be cooled, but with today’s electric rates where there are peak and off peak hours, many of us have the AC totally off during the peak hours and they come back on after peak, and I want the room cooled NOW, not in 2-3 hours.
For me the answer is to have a variable speed fan so the rooms will cool fast because lots of air is moving, and it can run slower and be less noisy after we get to a certain point. I also have an evap cooler for dryer times, and they do exactly this.
When designing my first low
When designing my first low-load home (Charlotte, circa 1998), I couldn’t get my mind around the resulting low face velocities and woefully inadequate register throws. My design cooling load was only 1.7 tons for 3,165 ft2 conditioned floor area (works out to 0.25 CFM per square foot @ 400 CFM/ton). At the time, I had not transitioned to mechanical design work and was befuddled as to how to reconcile such low airflow with conventional wisdom (from Manual T) regarding register throws and air mixing.
According to Hart & Cooley engineering tables, most of my diffusers would have throws in the range of only 3 to 4 feet. So I contacted 3 experts – Dave Fedders (technical guru at H&C, now retired), Rick Welguisz (airflow guru at Trane, now retired), and Hank Rutkowski, author of ACCA’s design manuals. All three said the same thing: With envelope loads that small, I needn’t worry about throws and air mixing. So I relaxed and did the best I could with what was available (I love H&C’s RoyalAire 531 floor diffusers). It all worked out. The house turned out to be very comfortable and very efficient (HVAC averaged ~$15/mo).
What I learned from that experience and confirmed many times since as a mechanical designer for low-load homes, is this: as we ratchet down envelope loads, air mixing becomes less and less important. When a low-load home is stable and relatively uniform, natural convection provides more than enough air mixing to achieve even temperatures. Similarly, there’s no need to extend branch runs to the perimeter (with rare exceptions). You just have to deliver the air to the room. And more generally, longer runtimes with right-sized equipment also contributes to air mixing.
After throwing stones at ACCA for several years for not addressing load load homes it ts design manuals, I was invited to participate in the (current) 3rd edition of Manual D (circa 2008). One contribution I made was to get Hank to address this dilemma, albeit in a small way, ergo Allison’s above-quoted citation from Appendix 15.
As an aside, ACCA’s Manual LLH (HVAC System Design for Low Load Homes), just published this year, is long overdue. One thing most folks don’t realize is that the term ‘low load’ now applies to virtually any home built and verified to meet today’s energy codes.
Wait — low velocity in a
Wait — low velocity in a duct increases the conduction losses (winter) and conduction gains (summer). This is very significant. This is the reason why many monitored tests of installing smaller air conditioners on the same duct system showed lower delivered efficiency and higher energy use — overwhelming the effect of gains from downsizing.
John, you’re getting ahead of
John, you’re getting ahead of things here. See the very last section of the article. And besides, low velocity doesn’t always imply higher conduction gains and losses. But that’s for the next article. (Also, I’m surprised you’re the first to mention this.)
John, you beat me to the
John, you beat me to the punch! And Allison, I suspected this would be covered in your follow-up article. I’m guessing you’ll mention locating the ducts in conditioned space, and deeply burying them if in attics, which will help mitigate conduction gains/losses. A couple of questions come to mind though: how do you determine the sweet spot in duct sizing? And is there a program (Elite?) that is capable of factoring in velocity? If there aren’t any such programs, how do you decide how much to adjust your calculations when designing a system? You don’t perform a manual calculation for every fitting do you?
Ha! Allison, I only read the
Ha! Allison, I only read the comments of this article to see WHEN someone would mention this.
Yeah, I should have mentioned
Yeah, I should have mentioned conduction losses but I haven’t designed a system with ducts outside the conditioned space in over a decade! I forget some builders still allow attic ducts =:-0
Well all the ducts are in the
Well all the ducts are in the conditioned envelope right 🙂 The nice slug of cold air coming out of a long unconditioned run in winter makes the following way too hot air soo much better.
One related topic that I haven’t wrapped my head around is how the number of downstream branches or trunk reducer effects TEL of a branch. I am just a homeowner that can stumble through the ACCA speed sheets, does Manual D explain this in detail?
Allison I believe you are
Allison I believe you are incorrect about the equivalent length of a fitting. f you reduce the airflow in a fitting from 900cfm to 450 cfm the resistance at 450 cfm will be about one quater of that at 900 cfm. But the effective/equivalent length will remain about the same.
The 75 percent drop in static pressure will still show in your calculation as you will be selecting a lower friction rate in manual D
Richard, Appendix 3 in Manual
Richard, Appendix 3 in Manual D explains how to account for this.
Richard, as Danny said,
Richard, as Danny said, Appendix 3 of Manual D explains this and Appendix 4 goes further with it. The former starts with this: “Some practitioners assume that the equivalent length of a fitting is an invariable and unconditional description of the aerodynamic efficiency of the fitting. This is not true!” If you haven’t read those two appendices, I encourage you to do so.
Regarding increased
Regarding increased conduction losses/gains with lower velocities, “This is very significant”. Yes I agree on slightly increased loads, but we must condition the ducts regardless of velocity, so that is a given. But “very significant”? I don’t see it yet. In a 100 lineal feet supply path, 900 fpm vs. 450 fpm adds about seven seconds to the duct sidewall exposure. And I insist this duct is in a conditioned area or well insulated.
But I will keep an open mind and look forward to the next article.
I am with you in that it is
I am with you in that it is hard to believe that conduction losses are a significant issue going between 900fpm to 450 fpm. Dropping velocity involves increasing duct size (surface area) and an increase in dwell time, but also a drop in the forced convection heat transfer coefficient.
There is a definite comfort issue when cycling and pushing out the slug of cold or hot air.
For high efficient homes, with ducts in the conditioned space, this is not an issue.
Dan — let us say your seven
Dan — let us say your seven seconds additional resident time is correct — what about the additional surface area needed to drop the velocity in half?
Great discussion. Before we
Great discussion. Before we start to do the math on surface area and conductive losses, I would like to reset to the real world. Truth is, when I am close to the fan, I cannot afford nor do I have the room to get much lower than 700 fpm. But 700 is my goal and this still makes a huge difference. My 85 EL is now down to about 50ish and if I can get to 600 fpm then my 85 EL gets below 40 EL! (Allison, are you checking my math?) But even at 700 fpm, I must use the good fittings. This is the science we need to teach.
Now shift to the 100 cfm branch run. I am already down to 500 fpm in my 6 inch round, so no larger ducts are needed. None of the ACCA EL images/ratings apply since they all assume 900 fpm. I do not worry too much about branch run fittings or 90s.
So the only changes we need to consider may be the middle stuff. My 400 cfm trunk portion should be a 10 x 10 instead of an 8 x 8. 600 fpm vs 900 fpm.
And that is the lesson for the real world. When you can, drop that velocity and very significantly reduce resistance. We really are not adding too much surface area, unless you had the whole system at 900 fpm in the original design. That’s the designer that does not understand he will drown using a soda straw for a snorkel!
So those of you who still
So those of you who still work in “normal” homes please tell me are the ducts now all really in the conditioned space or insulated to a level at least as high an R-value as the ceiling insulation?
If so I will have to agree that the problem has been solved: all the building codes in the US have been updated and all existing houses have been retrofitted to this “standard”.
On another note — I have to say the experimental data I am aware of shows that increased residence time and either longer run times or increased surface area make a significant difference. Specifically: a) downsizing ACs using the same duct system has shown mixed results averaging 0% savings; b)Mark Modera’s research was published in “Science and Technology for the Built Environment”. In one experiment he varied the amount of duct system operating under identical conditions and found unsurprisingly that increased ducting (read surface area) reduced the distribution efficiency from .78 to .40. Of course the increased losses depend on the amount of increase in area.
Remember for “those few duct systems that are still in the attic and not superinsulated” the duct system is a giant heat exchanger.
@John, for the majority of
@John, for the majority of the market (including new builds), I agree that conducted losses can be significant and must be accounted for in the design. Sadly the IECC has yet to mandate ducts inside conditioned space. When R8 ducts are routed through a hot unvented attic, it’s imperative to minimize the distribution system’s thermal footprint (i.e., both in terms of runout length and cross-sectional area).
But to the extent that low velocity (and thus inadequate throws) is forced upon designers due to unusually high floor-area-to-load ratios (above, say, 1500 ft2 per ton), which was one of the points of this article, conducted losses are pretty much a non-issue. In my experience, the inadequate throw dilemma only arises out of necessity in low-load homes with ducts inside the thermal envelope.
David, what do you mean by
David, what do you mean by “low velocity (and thus inadequate throws”? In this blog, I believe that Allison and others are talking about low velocity in the ducts, not at the supply register. So are you saying that low duct velocity results in inadequate throws? As Allison indicated, it is the supply register and connecting boot that determines flow, not the upstream duct velocity. To me, this is an important point since some companies tout the benefits of Small Duct High Velocity (SDHV) systems in terms of better room air distribution, but the two factors (high duct velocity, high throw velocity) are just not directly related. In my opinion, this is a major flaw in the proposed ACCA LLH Manual.
@Roy, true, low duct velocity
@Roy, true, low duct velocity doesn’t necessarily imply low face velocity, but when dealing with unusually high floor-area-to-load ratios, practical considerations (i.e., available diffuser sizes) limit the designer in terms of achieving recommended throw patterns, as Allison pointed out. My point was simply that throws become less and less important as we ratchet down envelope loads.
Low duct velocity can also result from design decisions that aren’t necessarily driven by tiny loads. For example, the designer may use larger ducts and fittings in order to reduce blower energy. This may or may not lead to throw issues, depending on design airflow and other factors.
Regarding SDHV… high velocity distribution deserves it’s own article, but suffice to say I’m not a fan. SDHV has limited application in retrofit (e.g., historic preservation projects) but it imposes far too high of an energy penalty to be a viable option for modern construction, with no upside, in my opinion.
Excellently expressed article
Excellently expressed article. This subject just came up in a class I conducted on reducing humidity by manipulating air volume and I was not able to properly defend that low velocity is rarely a concern. I need to read my emails sooner.
To the degree that we can use
To the degree that we can use this forum to express opinions contrary to ACCA “Standards” (and I hope this is true since they appear to pay no attention to objection comments to their proposed standards), Let me say: One of the major flaws with Manual D is the lack of including surface area in the calculations. They still allow the ducts to run all the way to the outside walls of a house with the ducts in the attic. Absurd.
The duct length as well as ELA should be minimized. Short Ducts Rule!!!
John,
John,
Although Manual D does discuss “Duct System Efficiency” in Appendix 10, it technically is not the best forum to address the question. Manual J is replete with references to the subject and even provides Worksheet G for the practitioner to be as accurate with real world duct load assessment as they want to be.
I don’t see ACCA as controlling design attributes. I see their tech pubs as giving guidance and recommendations. The creator of the design drives the bus. ACCA simply provides the necessary information to define the consequences of those decisions.
Sometimes interior distribution isn’t practical as with tray or cathedral ceilings that cannot provide the coanda effect and subjects the occupants to primary air in the occupied zone which is precluded from hitting the opposite wall.
Sometimes, the owner drives dumb decisions. What if Mr and Mrs Shallow Buyer want terminals over the windows instead of curved blades 2 ft off the interior wall??? Or for that matter, they insist on hardwood floor terminals to match the floor stain. Just yesterday, I had a homeowner insist they don’t want an exhaust fan over the cooktop. Can you believe that isn’t a code requirement?
Although we may not agree, America still is a place of $500 libraries and $50,000 kitchens. In other words, making stupid decisions is a protected civil right.
I also see and appreciate the years of hard work from Hank R and Glen H working within “committees”, with no input from those members simply wanting their name in the book. Then they must perform a balancing act between writing normative language, acceptable to the code police while never loosing sight of their directive to serve the contracting community with lucid and defensible scholarship that will hopefully save them from having to find an answer from ASHRAE’s Book of Fundamentals.
Lastly, did you notice my overt kindness and respect in not mentioning the “B” word in my post? :>)
I have read quite a few of
I have read quite a few of the comments in this thread, and have to say the problem I am incurring is outside the realm of what most of you are discussing, so I would like to put this out there. Most everyone is eluding to 450-900 fpm velocity. Well I am seeing duct systems with less than 200 fpm velocity. These homes are experiencing high humidity and comfort concerns. The duct systems/indoor units are installed in attics. I have already noted and explained the horrible under sizing of the ridge vents in most of these attics, not to mention some of the soffit vents that are covered up by blow in insulation. So the heat is more than readily available to transfer to the ducts due to this. The ducts are suspended in the attic, not buried in the attic insualtion, although I do like that idea. The second floors are doing better than the first floors, which the air is almost not existance coming out the vents on first floor. I am a firm beliver that too much of a good thing is a bad thing and grossly oversized duct work slows the air down way too much. I do understand and believe in less restriction is better to a point, the point of deminishing returns. Anyone seeing velocites below 300 fpm in trunks?? This is what I am fighting now.. Thanks for any input..
Unless this duct system is
Unless this duct system is leaky and/or not well insulated with a good vapor barrier, a low velocity due to oversized ducting can be corrected with balancing dampers. Since the second floor has better air flow, reduce the air going to the second floor outlets to allow more air to go to the further outlets on the first floor.
My guess is there is more of an issue than just low velocity.
@Richard, could you provide
@Richard, could you provide an example A/C tons, trunk airflow and dims? I personally have never seen trunk velocities that low. For example, to get to 200 FPM on a 3-ton system, assuming 1200 CFM, the trunk would have to have a cross-section of 864 sq.in. or roughly 30×30, or 33″ round! I can’t believe anyone would build that!
As John Proctor points out, such a huge duct surface area acts to increase conducted losses. In a hot attic, trunkis that size would greatly diminish system capacity even with code-mandated insulation.
Lastly, am I to understand you’re seeing a single system serving two floors? That’s no longer allowed in some states, at least not without automatic zone control. When the upstairs zone is off, the closed damper should push all (or most) of the air to the first floor, but the zones still need to be reasonably balanced against each other in order to maintain enough airflow to each zone when both zones are calling. On the other hand, without zone control in a multi-story home, all bets are off. At a minimum, the system would need to have a manual balancing damper to adjust the upstairs/downstairs balance from winter to summer.
I am not aware of any codes
I am not aware of any codes or regulations requiring separately controlled systems for multiple floors. Which states have such codes/regulations? It’s not required in my area and seems to be quite a restrictive code/regulation.
@Robin, I work nationwide and
@Robin, I work nationwide and I’ve encountered this several times over the years, although I can presently only recall one specific example: the North Carolina State Board of Examiners that licenses mechanical contractors requires that forced air systems in multi-level homes have thermostatic control on each level. This can either be accomplished with separate systems or automatic zone dampers. Perhaps John Proctor can cite others.
In any case, my point to Richard wasn’t that the home(s) he described might be in violation of such a regulation, but simply to give credence to my position that it’s poor design practice to condition multiple levels with a single zone system.
David, I agree 100% that
David, I agree 100% that multi-level buildings, whether residential or light commercial, should have levels controlled independently. More than any other aspect, different levels have completely different heat gain and heat loss factors that can vary within hours depending on whether the Sun is shining or not. North Carolina does have some of the most stringent HVAC codes, so that doesn’t surprise me.
I also agree with both you and Richard that mechanical zoning is much preferred over air zoning from a central system. I don’t do many installations these days, but my son and I just conditioned a friend of his 1850s brick construction mill house with three levels. We opted for using 2, 4 zoned ductless systems with the interior units zoned by levels more so than by geographical positioning of the house. In this way, we were able to keep the capacity of the equipment lower because the upper level uses most of the unit capacity in the cooling mode and the lower levels use more capacity in the heating mode. This type of system also controls the high humidity we have in the Mid-Atlantic area when there is not enough heat load to keep systems operating for long periods of time.
David — Good to hear from
David — Good to hear from you. As you are aware, I believe the idea of zoned systems even for multi-story homes is oversold. As a result people are putting in the infamous bypass. I am happy that you seemed to elude to capacity shifting which is moving some of the capacity from one zone to the other rather than “all the air” from one zone to the other. Capacity shifting allows good duct design, eliminates the awful bypass and enables a better controlled air flow through the entire system. There are complaints from contractors in states that have mandated zoned systems that they are seeing a lot more humidity problems (due the the common practice of installing a bypass) Check out this demonstration https://www.proctoreng.com/energy-efficiency/zones.html#video
@John, thanks for mentioning
@John, thanks for mentioning the ‘infamous’ bypass issue. Poor humidity control is but one of several detrimental consequences of routing supply air directly back into the return side.
And of course, zoned systems can be designed without a bypass. In fact, at least one manufacturer, Carrier, prohibits the use of bypass with its Infinity Zone Control System.
The problem I’m running into with beyond-code homes is that the loads may be too small, even for mini-splits, to use equipment zoning and, as you know, the only way to maintain proper temperature control per level from winter to summer is to have thermostatic control on each level. Seasonal manually operated balancing dampers are a poor substitute, IMO.
Good morning all, thanks for
Good morning all, thanks for the responses to my post. Just to put the information into perspective as I have noticed people mentioning North Carolina and the codes in North Carolina. I am actually in North Carolina and have lived/worked here my whole career.
The homes I am having these issues with are built to Energy Star type standards.
The attics are atrocious, minimal ridge venting and soffit venting is not that great either. I have addressed this with builder and upper management. Attics in these homes get very hot and humid, not to mention stagnant.
Also, these homes are slab homes that sit right on grade, and I am aware and have pointed out that drainage is an issue. I am not that great with building code, but understand and follow mechanical codes pretty closely. I was always of the understanding that these slab homes were supposed to be built in a fashion to promote drainage, either graded up to slab or grade yard down. These yards are as flat as a piece of paper, I even have pictures of me standing in 1-2” of standing condensate water to assess one outdoor unit.
These are 1 system 2 zone homes, which is permitted in NC. Indoor units are located in the attic along with most of the duct work that is R-8 in attic and switches over to the permitted R-4.2 once it goes inside the envelope.
Manual D states they want to see 700-900 FPM in supply trunks, and 600-700 FPM in return trunks. I classify my trunks as the plenums and the larger/zoned ducts feeding the distribution boxes for each zone. Yes, this is a box and flex system, that is how new construction is done, cheap, cheap, cheap…. Not that I agree with it.
In my supply plenums I am seeing anywhere from 794.5 on the high end down to 620 on the low end. Once the air leaves the plenums and enters the larger flex trunks, the velocity averages 483.3 on the high end to 235.5 on the low end.
In my return plenums I am seeing anywhere from 544.3 on the high end down to 281.0 on the low end. Before the air enters the plenums from the flexes, I am seeing 559.5 on the high end down to 198.3 on the low end.
My velocity measurements were conducted by traversing each duct with a hot wire anemometer with the correct duct dimensions put in before each test, just in case anyone is questioning testing practice.
The person responsible for sizing/designing these systems and I have spoken at length. According to his designs, the systems are within the ACCA Manual S sizing restrictions of 90-130% I believe it is. The refrigeration sides are running in the 115% range of load, to allow for less strip heating in the winter. I struggle to agree with this sizing as per customer discussions, they were maintaining 70-72* indoor temp with high humidity during our 96-100* this summer. Maintaining temperature but having high humidity are the classic signs of oversized equipment.
I do not think that low low velocity is the only problem I am dealing with. But it is a compounding factor to go with the permitted oversizing, lack of grade and water getting back under the slabs, wicking up back into house, shoddy craftsmanship on attic ventilation, over sizing of equipment, home construction in general… But low velocity with over sized duct work in extremely hot attics increasing the conduction surface, in my mind is something we can control. No need to add to the issues..
Oh and the systems range from 2.5 ton to 4 ton…
I hope I was able to give more of the pertinents that people were questioning in this response. Any ideas/input is greatly appreciated. Thanks
Richard, I still do not
Richard, I still do not believe low air velocity is a significant part of your issues. It sounds like oversized cooling is definitely an issue, but reduced air volume will actually allow the dehumidification to be better as the indoor coil becomes colder, faster, and stays below dewpoint.
It sounds like you already have control between levels through zoning dampers.
Low duct velocity, in of
Low duct velocity, in of itself, has no impact on latent performance. In particular, low velocity may be indicative of low airflow across the evaporator coil, which would actually *increase* moisture removal capacity.
The Manual D velocity references you cite are from Table A1-1, Air Velocities for Noise Control. So it should be obvious from the title that these values are intended as upper limits (“conservative” and “maximum”). There is no lower limit for return-side velocity, and I would argue there’s no lower limit on supply-side velocity, at least not within the scope of Manual D. (That’s not to say there’s never a downside associated with low supply-side velocity, as noted in the article and some of the comments).
The other issues you mention seem like potential contributors to high RH, but these topics are beyond the scope of this article. I suggest that you seek advice at the Home Energy Pros Forum.
Richard. The moisture that is
Richard. The moisture that is removed from the house by the air conditioner is done at the evaporator coil. It is not done in the duct system. How many CFM/ton is moving through the evaporator coil? The velocity of the air through the ducts will effect the delivery temperature of the air, but not the amount of moisture in the supply air.
I believe you have a zone
I believe you have a zone damper stuck in the middle. It would normally be a higher velocity when conditioning either upper or lower levels but when trying to do both it is inadequate.
Also check for blockages at the evap coil . It could be heavily impacted.
John Proctor, I am curious
John Proctor, I am curious about something that is probably a sidebar to this discussion. But I would like to know your thoughts.
I have reviewed your scholarship and watched the videos on the bypass damper being used (and usually abused) in zoning systems. AND, I dont think anyone can argue that there is an energy penalty for dumping unrestricted conditioned air back into the return of a cooling system.
However, what other negatives do you think are applicable to using a properly designed bypass damper as prescribed in ACCA Manual ZR. ZR limits the amount of bypass air based on the OEM’s Leaving Dry Bulb (LDB) limit? Worst case scenarios I have seen even with close to 40% bypass, the LDB still hovers around 44 degrees F. Of course that is in my climate at 90 degree F outside, 75 degree F inside with 50% RH. Of course all bets are off with the surgeon who insists on 68 degree F indoors. Contrary to his belief, the laws of physics still control.
Now that we’ve went through
Now that we’ve went through this exercise let’s throw low temp air into the equation, then the throw and churn of the air really matter. Saw you discharge air temp is 40 deg off of a chilled water coil? You want some higher velocities in that case through your duct. But you can have smaller ductwork since you are gaining more tonnage out of your system because of the lower discharge air temps. Give me your thoughts on that please. Thanks
@Darin, you seem to be
@Darin, you seem to be conflating coil face velocity and duct velocity when the two are (or at least should be) independently determined by design. Supply air temperature is a function of the volume (CFM) of air that moves across the coil. For a given system, airflow, and operating conditions, coil geometry (in particular, face area) determines supply air temperature, which is unaffected by downstream duct & diffuser geometry. Thus, duct velocity has no bearing on system capacity (notwithstanding any impact on conducted heat transfer through duct surfaces).